acespicoli
Well-known member
English version
![]()
Bread from Stones by Julius Hensel– Book Review
Bread from Stones “Our most optimistic expectations are no less than the realization of an old dream: ‘What will fertilizing with stone dust accomplish? It will turn stones into bread, make barren regions fruitful, and feed the hungry.’” --Ward Chesworth and coauthors, quoting Julius Hensel...www.remineralize.org

Agrominerals - Wikipedia
Read the book

https://drive.google.com/file/d/1P7xV8QFoa79BObiV7ZDcZLhlz9JcvI-5/view?usp=sharing
A poster here in this thread had mentioned to add glacial rock dust once
Also greensand is already part of the fertilizer and river sand I have some clay sorbent im considering trying

will review the rock fertilizer recipes in the book as time goes on and leave results
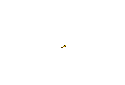
Agrominerals very brilliant my PHD friend

Last edited: