acespicoli
Well-known member
Tetraploid monoecious hemp plants are selected by examination of the
number and size of stomata, the number of epidermis and stoma cells,
the size of pollen grains, and their number of pores. (19)
selecting tetraploid males
Polyploids are valuable for their genetic diversity, but they are unpredictable and usually are
unstable in the first generation.
They also tend to be sterile and must be propagated by clonal cuttings to be useful for
subsequent breeding.
2nd 3rd Gens pheno hunt
Studies by Warmke and Zhatov revealed that the normal sex ratio for diploids (2n) is nearly 1:1, but tetraploids (4n) form a new class (XXXY) and develop about 7.5 females:1 male, plus female-hermaphrodites. The XXXX is female; XXXY is female-hermaphroditic; XYYY is male-hermaphroditic, and YYYY is male. The XY determination of sex does not account, however, for the development of some monoecious strains. Seemingly, the sexual expression of hemp can be controlled by some other gene set(s) influencing different aspects of flowering. Environmental conditions also can overpower the genetic expression of Cannabis' gender, especially in the final stages of flower production. (20-23)
Female inflorescences of three marijuana strains grown under commercial conditions were visually examined at weekly intervals. Beginning around week 4 of the flowering period, the appearance of individual anthers or clusters of anthers within the bract tissues adjacent to the stigmas was observed in hermaphroditic flowers at a frequency of 5–10% of the plants examined (Figures 2A–D). The anthers were visible in weeks 4–7 of the flowering period and were present until harvest. In rare instances, the entire female inflorescence was converted to large numbers of clusters of anthers (Figure 3). Scanning electron microscopic examination of the stigmas that were present in hermaphroditic flowers showed the papillae (stigmatic hairs) (Figure 4A), which in mature inflorescences originated from a central core (Figure 4B). Individual anthers that were produced in hermaphroditic inflorescences were shown to consist of an outer wall (epidermis and endothecium) with a longitudinal groove (stomium) (Figure 4C) which, upon maturity, expanded and dehisced to release pollen grains (Figure 4D). Bulbous structures presumed to be trichomes were also observed forming along the stomium of the anther (Figure 4E). When viewed under the light microscope, the anther wall and stomium could be seen and pollen grains were released into the water used to mount the sample (Figures 5A–C). Some pollen grains had collapsed when viewed under the scanning electron microscope (Figure 5D). Pollen germination was observed within 48–72 h on water agar and ranged from 10 to 30% (Figure 8A).
FIGURE 4
Figure 4. Scanning electron microscopy of the stigmas and anthers in hermaphroditic flowers of Cannabis sativa. (A) Young developing stigma with receptive papillae or stigmatic hairs (arrow). (B) Older stigma in which the stigmatic hairs are coiled and collapsed around a central core. (C) Individual anther prior to dehiscence showing an outer epidermis with the beginning of a longitudinal groove (stomium) (arrow). (D) Mature anther that has dehisced and revealing pollen grain release (arrow). (E) Enlarged view of the stomium showing formation of bulbous trichomes (arrow) forming in the groove.
FIGURE 5
Figure 5. Light and scanning electron microscopic observations of anthers and pollen grains in hermaphroditic flowers of Cannabis sativa. (A) The anther wall and groove are visible and pollen grains can be seen packed within the anther pollen sacs (arrow). (B) Release of pollen grains into water used to mount the sample. (C,D) Intact and collapsed pollen grains as viewed in the light microscope (C) and the scanning electron microscope (D).
FIGURE 6
Figure 6. Flower and pollen development in genetically male plants of Cannabis sativa. (A–C) Male flowers formed in clusters at leaf axils. Each flower is pedicillate, with individual stalks. (D–F) Opening of male flowers to reveal 5 green-white tepals which expose 5 stamens each attached to a filament that dangles the anther. (G) Large amounts of pollen (arrow) being released through the longitudinal groove (stomium) of the anther. (H) Enlarged view of the stomium showing formation of bulbous trichomes (arrow) forming in the groove of the anther. (I) Close-up of a trichome with a short stalk (arrow). Pollen grains can be seen in the foreground.
In genetically male plants, anthers were produced within clusters of staminate flowers that developed at leaf axils (Figures 6A–C) at around 4 weeks of age. At flower maturity in weeks 4–6, anthers dangled from individual flowers and were observed to release large amounts of pollen grains, which were deposited in yellow masses on the leaves below (Figures 6D–F, 7). Such prolific release of pollen was not observed from the hermaphrodite flowers. Scanning electron microscopic examination of the anthers produced on staminate plants showed the release of pollen grains (Figure 6G). Along the longitudinal groove or stomium, the formation of a line of bulbous trichomes (Figure 6H) that developed on a short pedicel (Figure 6I) was observed, similar to that seen in hermaphroditic flowers. When pollen from male plants was deposited onto female inflorescences (Figure 8B) and viewed at 72–96 h, various stages of pollen germination and germ tube development were observed (Figures 8C–F).
FIGURE 7
Figure 7. Comparative growth of male (M) and female (F) plants of C. sativa strain “Blue Deity,” showing the more rapid growth of male plants to achieve taller slender plants that shed pollen onto shorter slower developing female plants. Plants originated from one seed batch produced from cross-fertilization that yielded male and female plants in approximately equal ratios. Seeds were planted at the same time and grown under a 24 h photoperiod for 4 weeks.
FIGURE 8
Figure 8. Light and scanning electron micrographs of pollen germination in Cannabis sativa. (A) Pollen germination in water after 72 h showing germ tube formation at a 20% frequency. (B) Female inflorescence showing protruding receptive stigmas. The flower heads were excised and pollinated in vitro using pollen collected from a male flower. (C–F) Pollen germination and germ tube development on stigmatic papillae in situ. Arrows show pollen grains in (C,D) and germ tube growth in (E,F).
Within the hermaphroditic inflorescences in which anthers were found, seed set was initiated, and mature seeds were observed prior to the harvest period (Figures 9A,B). From each of 3 inflorescences bearing seeds, a total of 34, 48, and 22 seeds were obtained. The seeds were removed and placed in moist potting medium where they germinated at a rate of 90–95% within 10–14 days to produce seedlings (Figures 9C,D).
FIGURE 9
Figure 9. Seed formation within hermaphroditic inflorescences of Cannabis sativa. (A) Longitudinal section cut through the female inflorescence showing outer protruding stigmas and unfertilized ovules. (B) Seed formation within a hermaphroditic inflorescence after 3–4 weeks. Some of the calyx tissue was cut away to reveal the underlying seeds. (C) Seeds recovered from hermaphroditic flowers, ranging from mature (brown) to immature (yellowish-green). (D) Stages of seed germination after placement in a cocofibre:vermiculite potting medium and incubation for 10 days.
PCR analysis was used to identify specific bands which correlated with the male or female phenotype in commercial marijuana strains. Seedling tissues from strains “Moby Dyck” and “Blue Deity,” produced through cross-fertilization by a commercial seed producer, showed a band size of approximately 540 bp in female plants, while two bands (ca. 540 and 390 bp in size) or one band (390 bp), were observed in male plants. The resulting ratio of male:female plants in seeds derived from these latter strains was 5:7 and 9:5, respectively (Figures 10A,B). In a third strain “Healer,” however, which were seeds obtained from outdoor cultivation of marijuana, only two male plants were identified among 16 plants; the remaining 14 were female (Figure 10C). By comparison, seeds obtained from hermaphroditic inflorescences of strains “Moby Dyck” and “Space Queen” yielded seedlings that all showed the 540 bp band size corresponding to the female phenotype (Figures 10D,E); the male-specific 390 bp band was absent. PCR analysis of DNA isolated from anther tissues (A) from hermaphroditic plants showed that the banding pattern was of the 540 bp band (Figure 10F). In contrast, the banding pattern observed in staminate flower tissues showed the 390 bp band (data not shown).
FIGURE 10
Figure 10. PCR analysis to identify male and female seedlings of Cannabis sativa. In female plants, a band of approximately 540 bp in size was observed, while in male plants, a 390 bp size band was always observed and the 540 bp band was sometimes detected. (A,B) Strain “Moby Dyck” and “Blue Deity” showed a 5:7 and 9:5 ratio of male (M) and female (F) plants, respectively, from seeds derived from a male:female cross. (C) Strain “Healer” showed a 2:14 ratio of male:female plants. (D,E) All female plants derived from seeds resulting from hermaphroditic flowers of strains “Moby Dyck” (D) and “Space Queen” (E). (F) PCR analysis of anther tissues (A) showing female composition compared to male (M) and female (F) plants. Water control with no DNA (C) and 1 kb DNA ladder (NEB Quick-Load®) (L).
Tetraploid-produced pollen was larger on average than diploid-produced pollen. A study in the Australian grass Themeda triandra revealed similar results with the diploids producing pollen significantly smaller than the tetraploid (Godfree et al., 2017)
William Blake.
To see a World in a Grain of Sand.
And a Heaven in a Wild Flower.
Hold Infinity in the palm of your hand.
number and size of stomata, the number of epidermis and stoma cells,
the size of pollen grains, and their number of pores. (19)
selecting tetraploid males
Polyploids are valuable for their genetic diversity, but they are unpredictable and usually are
unstable in the first generation.
They also tend to be sterile and must be propagated by clonal cuttings to be useful for
subsequent breeding.
2nd 3rd Gens pheno hunt
Studies by Warmke and Zhatov revealed that the normal sex ratio for diploids (2n) is nearly 1:1, but tetraploids (4n) form a new class (XXXY) and develop about 7.5 females:1 male, plus female-hermaphrodites. The XXXX is female; XXXY is female-hermaphroditic; XYYY is male-hermaphroditic, and YYYY is male. The XY determination of sex does not account, however, for the development of some monoecious strains. Seemingly, the sexual expression of hemp can be controlled by some other gene set(s) influencing different aspects of flowering. Environmental conditions also can overpower the genetic expression of Cannabis' gender, especially in the final stages of flower production. (20-23)
Hermaphrodite Inflorescence Development
Female inflorescences of three marijuana strains grown under commercial conditions were visually examined at weekly intervals. Beginning around week 4 of the flowering period, the appearance of individual anthers or clusters of anthers within the bract tissues adjacent to the stigmas was observed in hermaphroditic flowers at a frequency of 5–10% of the plants examined (Figures 2A–D). The anthers were visible in weeks 4–7 of the flowering period and were present until harvest. In rare instances, the entire female inflorescence was converted to large numbers of clusters of anthers (Figure 3). Scanning electron microscopic examination of the stigmas that were present in hermaphroditic flowers showed the papillae (stigmatic hairs) (Figure 4A), which in mature inflorescences originated from a central core (Figure 4B). Individual anthers that were produced in hermaphroditic inflorescences were shown to consist of an outer wall (epidermis and endothecium) with a longitudinal groove (stomium) (Figure 4C) which, upon maturity, expanded and dehisced to release pollen grains (Figure 4D). Bulbous structures presumed to be trichomes were also observed forming along the stomium of the anther (Figure 4E). When viewed under the light microscope, the anther wall and stomium could be seen and pollen grains were released into the water used to mount the sample (Figures 5A–C). Some pollen grains had collapsed when viewed under the scanning electron microscope (Figure 5D). Pollen germination was observed within 48–72 h on water agar and ranged from 10 to 30% (Figure 8A).
FIGURE 4
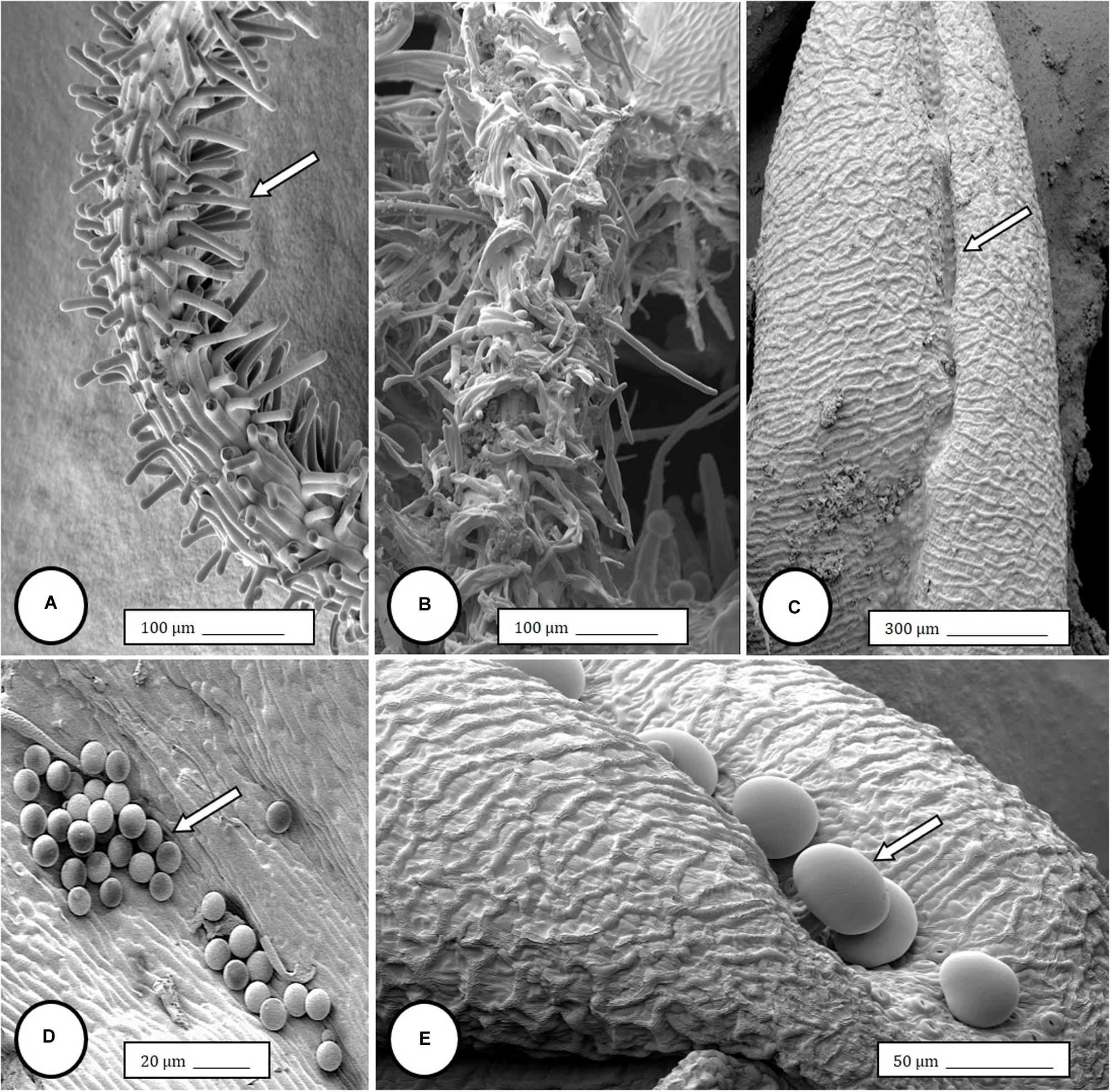
Figure 4. Scanning electron microscopy of the stigmas and anthers in hermaphroditic flowers of Cannabis sativa. (A) Young developing stigma with receptive papillae or stigmatic hairs (arrow). (B) Older stigma in which the stigmatic hairs are coiled and collapsed around a central core. (C) Individual anther prior to dehiscence showing an outer epidermis with the beginning of a longitudinal groove (stomium) (arrow). (D) Mature anther that has dehisced and revealing pollen grain release (arrow). (E) Enlarged view of the stomium showing formation of bulbous trichomes (arrow) forming in the groove.
FIGURE 5
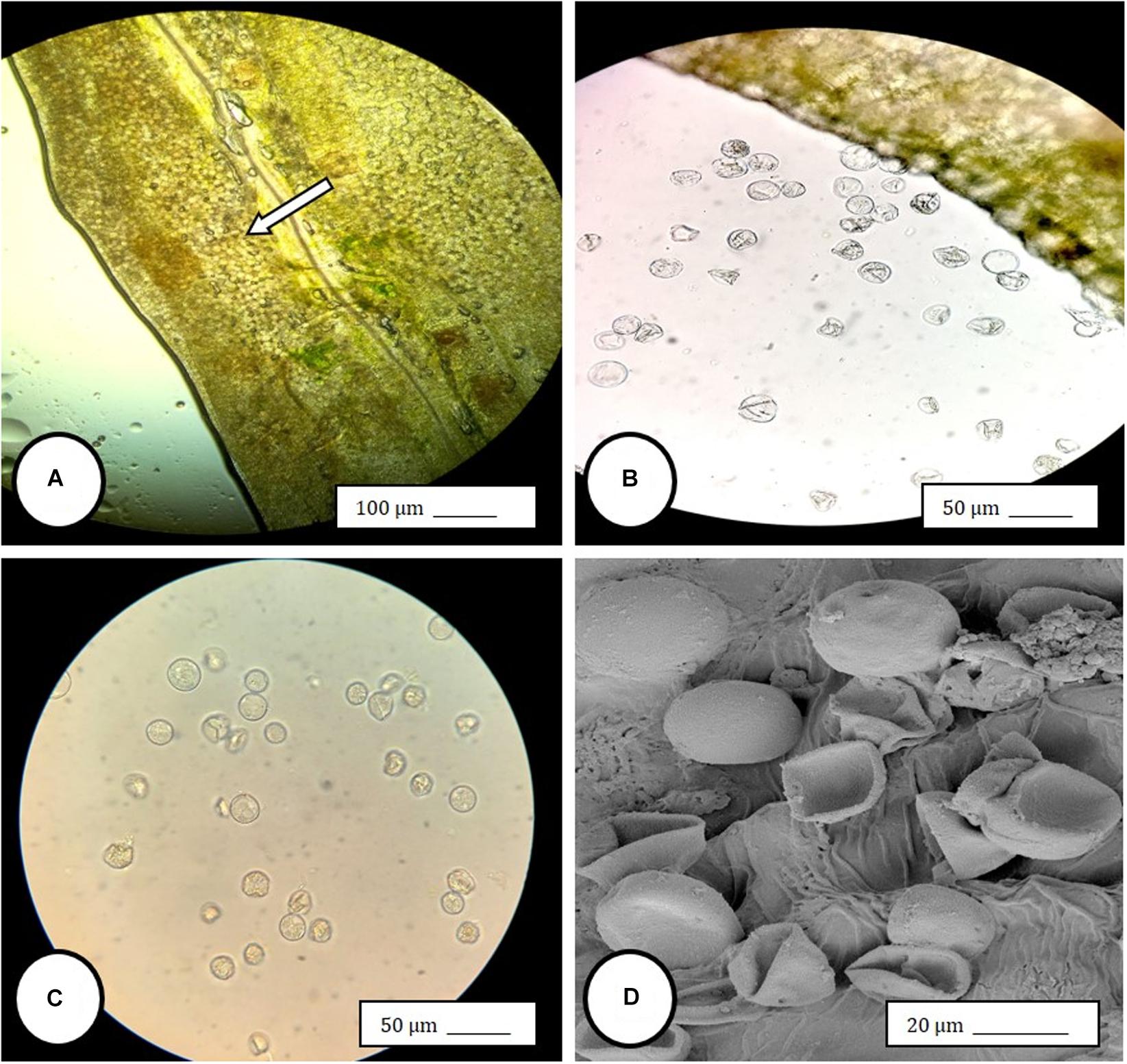
Figure 5. Light and scanning electron microscopic observations of anthers and pollen grains in hermaphroditic flowers of Cannabis sativa. (A) The anther wall and groove are visible and pollen grains can be seen packed within the anther pollen sacs (arrow). (B) Release of pollen grains into water used to mount the sample. (C,D) Intact and collapsed pollen grains as viewed in the light microscope (C) and the scanning electron microscope (D).
FIGURE 6
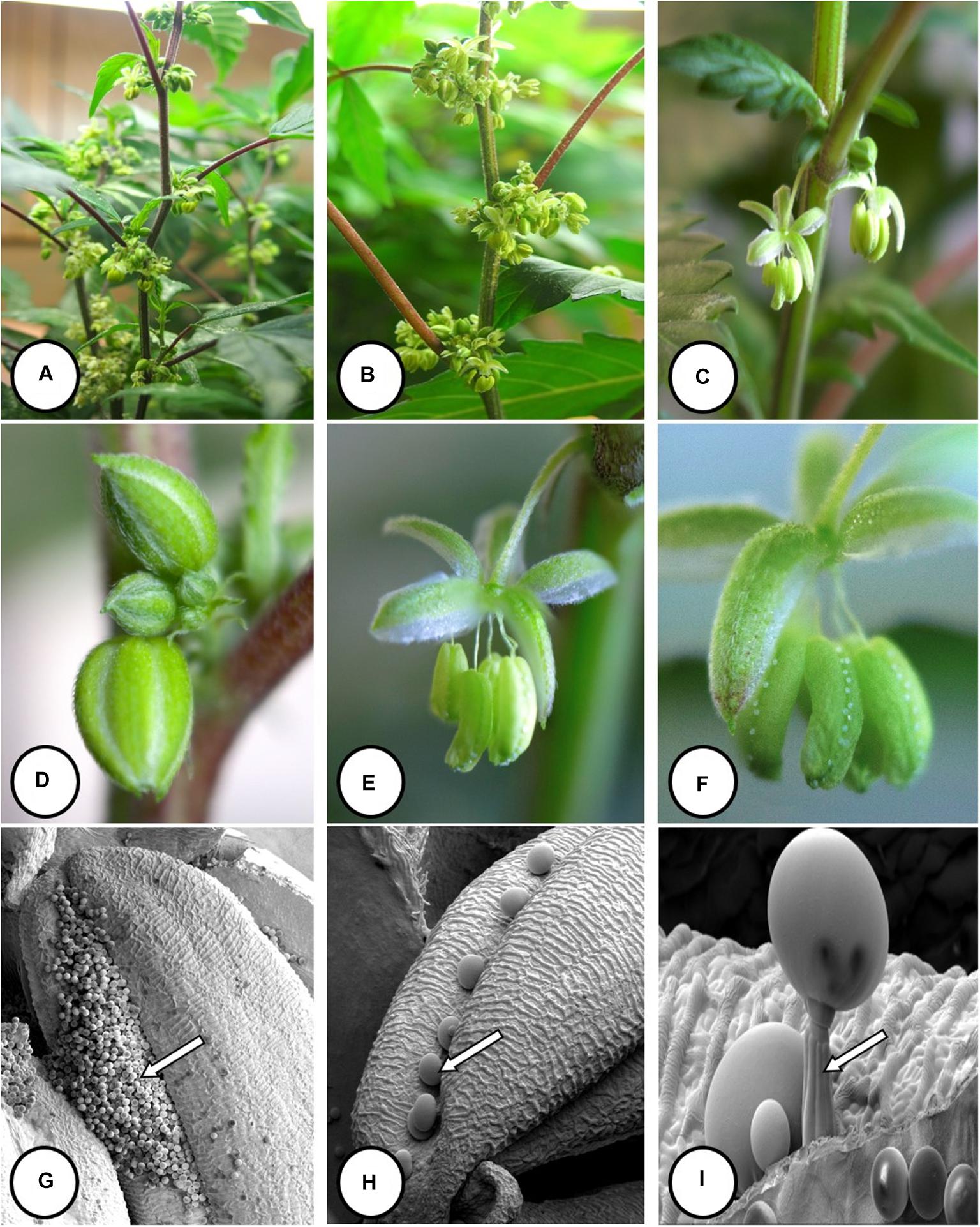
Figure 6. Flower and pollen development in genetically male plants of Cannabis sativa. (A–C) Male flowers formed in clusters at leaf axils. Each flower is pedicillate, with individual stalks. (D–F) Opening of male flowers to reveal 5 green-white tepals which expose 5 stamens each attached to a filament that dangles the anther. (G) Large amounts of pollen (arrow) being released through the longitudinal groove (stomium) of the anther. (H) Enlarged view of the stomium showing formation of bulbous trichomes (arrow) forming in the groove of the anther. (I) Close-up of a trichome with a short stalk (arrow). Pollen grains can be seen in the foreground.
Male Inflorescence Development
In genetically male plants, anthers were produced within clusters of staminate flowers that developed at leaf axils (Figures 6A–C) at around 4 weeks of age. At flower maturity in weeks 4–6, anthers dangled from individual flowers and were observed to release large amounts of pollen grains, which were deposited in yellow masses on the leaves below (Figures 6D–F, 7). Such prolific release of pollen was not observed from the hermaphrodite flowers. Scanning electron microscopic examination of the anthers produced on staminate plants showed the release of pollen grains (Figure 6G). Along the longitudinal groove or stomium, the formation of a line of bulbous trichomes (Figure 6H) that developed on a short pedicel (Figure 6I) was observed, similar to that seen in hermaphroditic flowers. When pollen from male plants was deposited onto female inflorescences (Figure 8B) and viewed at 72–96 h, various stages of pollen germination and germ tube development were observed (Figures 8C–F).
FIGURE 7
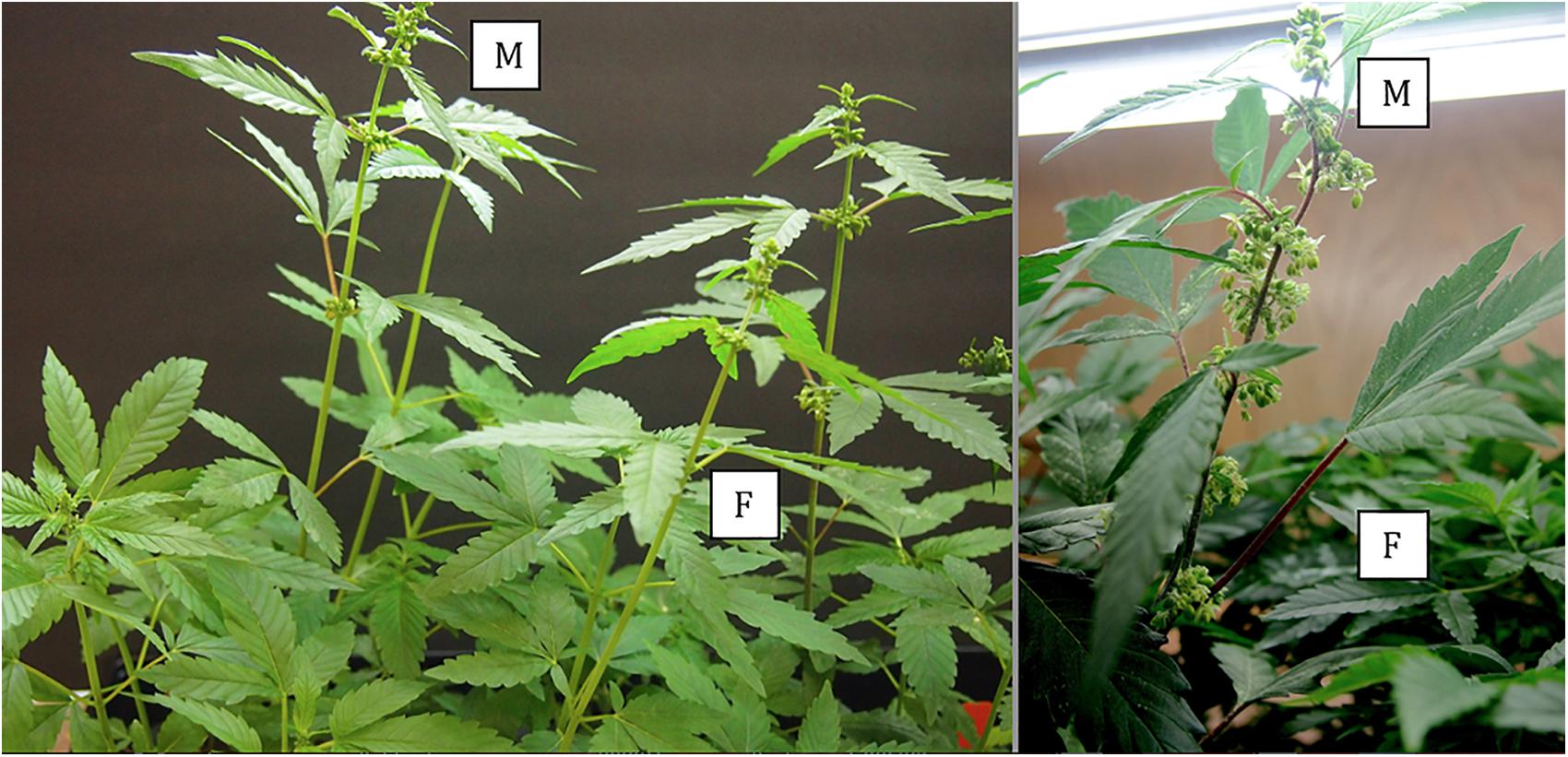
Figure 7. Comparative growth of male (M) and female (F) plants of C. sativa strain “Blue Deity,” showing the more rapid growth of male plants to achieve taller slender plants that shed pollen onto shorter slower developing female plants. Plants originated from one seed batch produced from cross-fertilization that yielded male and female plants in approximately equal ratios. Seeds were planted at the same time and grown under a 24 h photoperiod for 4 weeks.
FIGURE 8
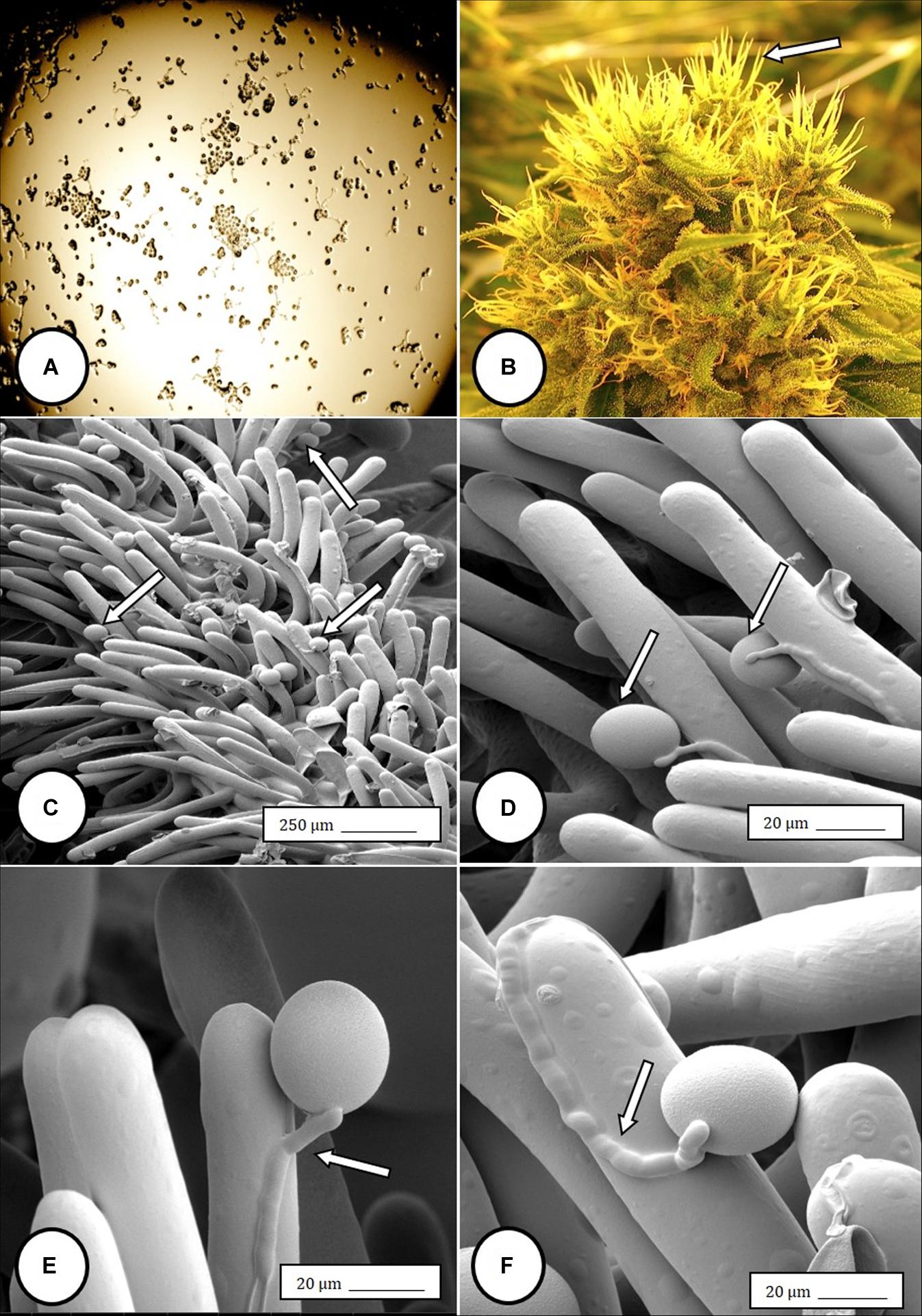
Figure 8. Light and scanning electron micrographs of pollen germination in Cannabis sativa. (A) Pollen germination in water after 72 h showing germ tube formation at a 20% frequency. (B) Female inflorescence showing protruding receptive stigmas. The flower heads were excised and pollinated in vitro using pollen collected from a male flower. (C–F) Pollen germination and germ tube development on stigmatic papillae in situ. Arrows show pollen grains in (C,D) and germ tube growth in (E,F).
Within the hermaphroditic inflorescences in which anthers were found, seed set was initiated, and mature seeds were observed prior to the harvest period (Figures 9A,B). From each of 3 inflorescences bearing seeds, a total of 34, 48, and 22 seeds were obtained. The seeds were removed and placed in moist potting medium where they germinated at a rate of 90–95% within 10–14 days to produce seedlings (Figures 9C,D).
FIGURE 9
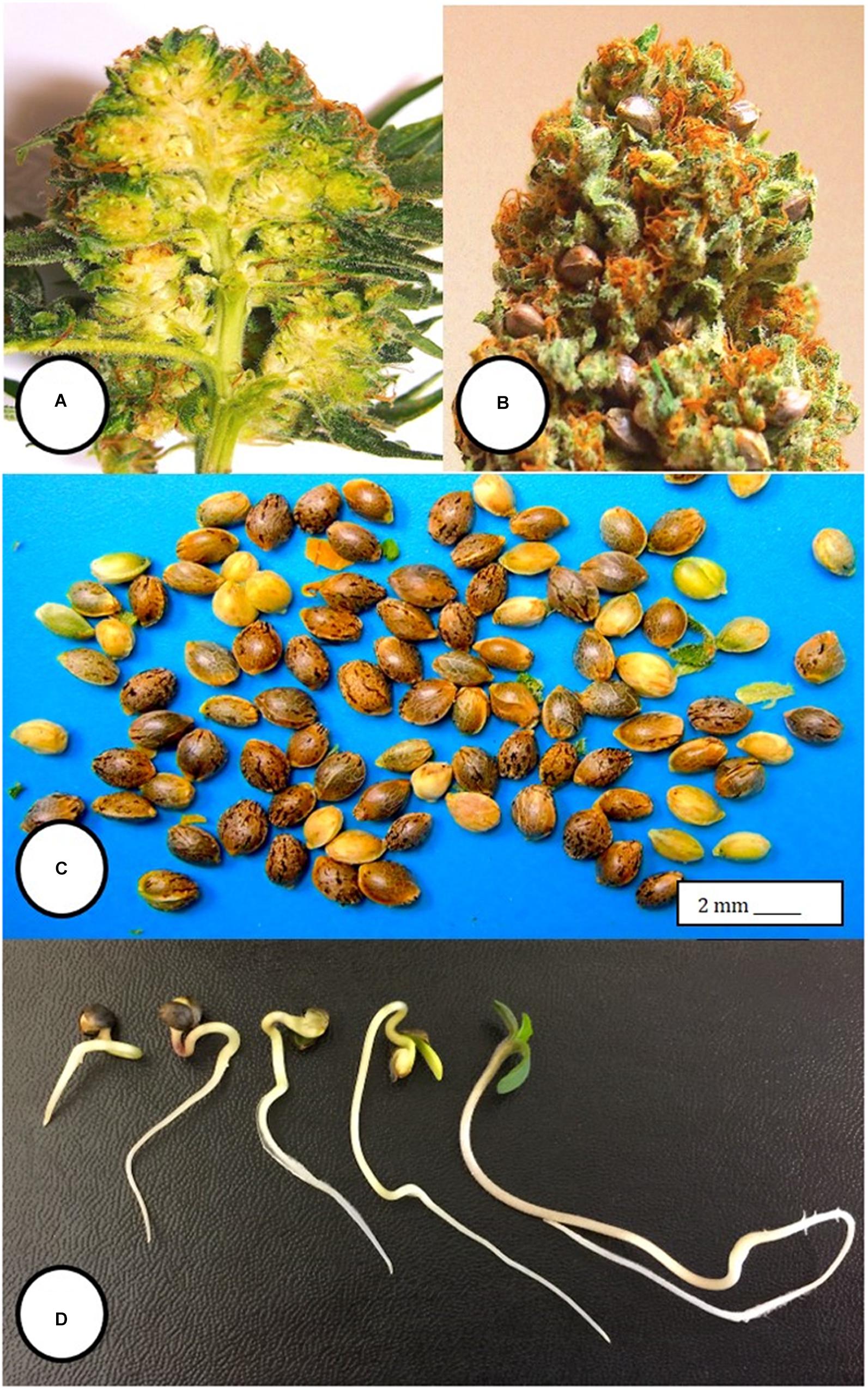
Figure 9. Seed formation within hermaphroditic inflorescences of Cannabis sativa. (A) Longitudinal section cut through the female inflorescence showing outer protruding stigmas and unfertilized ovules. (B) Seed formation within a hermaphroditic inflorescence after 3–4 weeks. Some of the calyx tissue was cut away to reveal the underlying seeds. (C) Seeds recovered from hermaphroditic flowers, ranging from mature (brown) to immature (yellowish-green). (D) Stages of seed germination after placement in a cocofibre:vermiculite potting medium and incubation for 10 days.
PCR and Sequence Analysis
PCR analysis was used to identify specific bands which correlated with the male or female phenotype in commercial marijuana strains. Seedling tissues from strains “Moby Dyck” and “Blue Deity,” produced through cross-fertilization by a commercial seed producer, showed a band size of approximately 540 bp in female plants, while two bands (ca. 540 and 390 bp in size) or one band (390 bp), were observed in male plants. The resulting ratio of male:female plants in seeds derived from these latter strains was 5:7 and 9:5, respectively (Figures 10A,B). In a third strain “Healer,” however, which were seeds obtained from outdoor cultivation of marijuana, only two male plants were identified among 16 plants; the remaining 14 were female (Figure 10C). By comparison, seeds obtained from hermaphroditic inflorescences of strains “Moby Dyck” and “Space Queen” yielded seedlings that all showed the 540 bp band size corresponding to the female phenotype (Figures 10D,E); the male-specific 390 bp band was absent. PCR analysis of DNA isolated from anther tissues (A) from hermaphroditic plants showed that the banding pattern was of the 540 bp band (Figure 10F). In contrast, the banding pattern observed in staminate flower tissues showed the 390 bp band (data not shown).
FIGURE 10
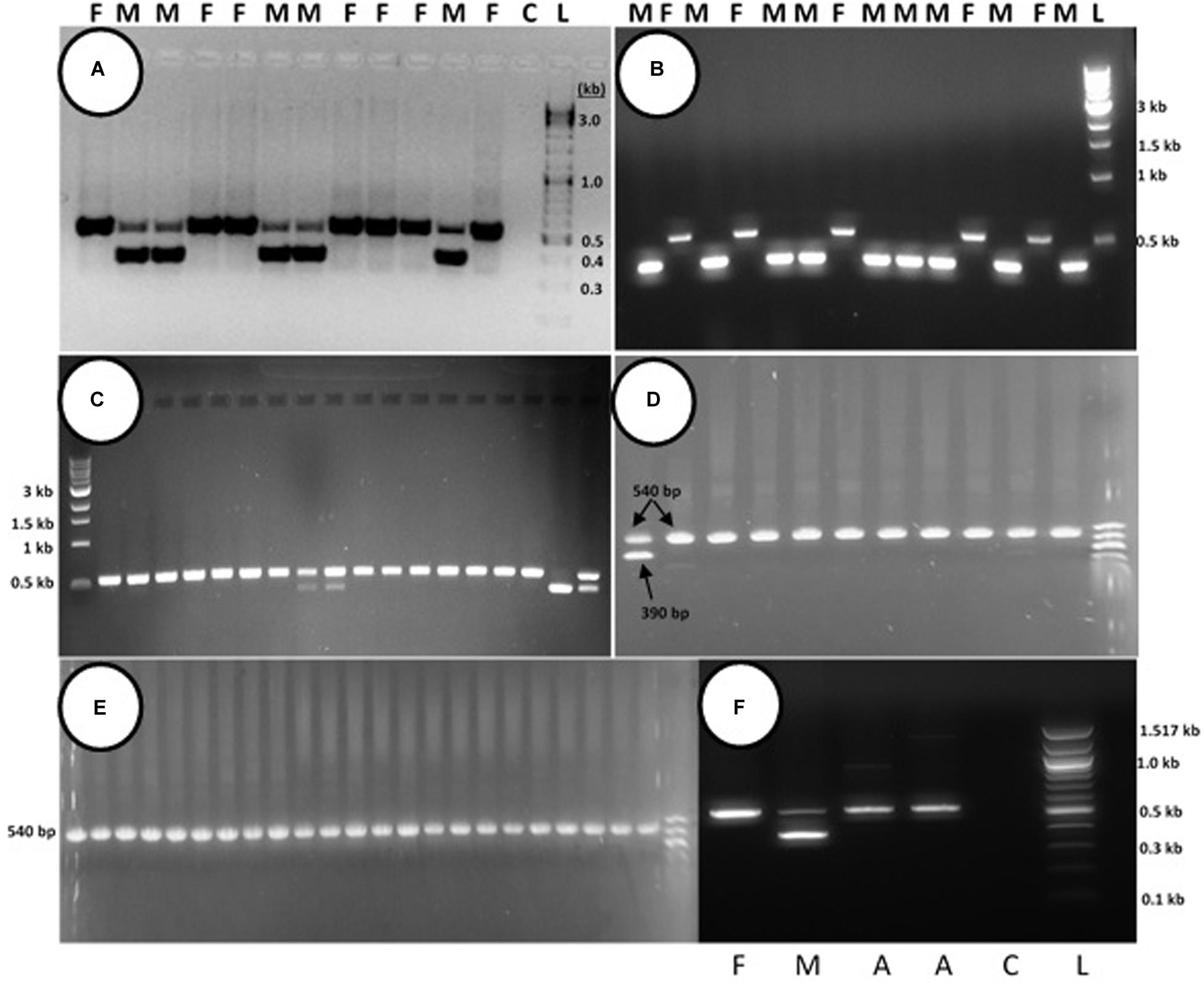
Figure 10. PCR analysis to identify male and female seedlings of Cannabis sativa. In female plants, a band of approximately 540 bp in size was observed, while in male plants, a 390 bp size band was always observed and the 540 bp band was sometimes detected. (A,B) Strain “Moby Dyck” and “Blue Deity” showed a 5:7 and 9:5 ratio of male (M) and female (F) plants, respectively, from seeds derived from a male:female cross. (C) Strain “Healer” showed a 2:14 ratio of male:female plants. (D,E) All female plants derived from seeds resulting from hermaphroditic flowers of strains “Moby Dyck” (D) and “Space Queen” (E). (F) PCR analysis of anther tissues (A) showing female composition compared to male (M) and female (F) plants. Water control with no DNA (C) and 1 kb DNA ladder (NEB Quick-Load®) (L).
Tetraploid-produced pollen was larger on average than diploid-produced pollen. A study in the Australian grass Themeda triandra revealed similar results with the diploids producing pollen significantly smaller than the tetraploid (Godfree et al., 2017)

Cytological observation of pollen development in diploid and autotetraploid rice hybrids. A to D, Normal pollen development in diploid rice. A, Dyad stage. B, Tetrad stage. C, Late-stage microspore. D, Bicellular pollen stage. E to L, Abnormal pollen development in diploid hybrids. E, Abnormal dyad stage. F and G, Abnormal tetrad stage (arrows indicate abnormal tetrad cells). H to J, Ex- amples of abnormal early-stage microspores. K, Abnormal midstage bicellular pollen (arrow indicates small pollen). L, Mature pollen stage (arrows indicate small pollen). M to X, Abnormal pollen development in autotetraploid rice hybrids. M, Abnormal dyad stage. N to P, Abnormal tetrad stage. Q, Abnormal early-stage microspore (arrows). R, Middle-stage abnormal microspore (arrow). S, Abnormal late-stage microspore (arrows indicate multiple apertures). T, Early-stage bicellular pollen (arrow indicates small pollen). U to X, Late- stage bicellular pollen (arrows indicate small pollen). Bars = 40 m
William Blake.
To see a World in a Grain of Sand.
And a Heaven in a Wild Flower.
Hold Infinity in the palm of your hand.
Last edited: