The THCAS and CBDAS share a high sequence similarity of 83% based on which a common ancestor was suggested initially (Taura et al., 2007b). Upon analysis of sequence variants of CBDAS and THCAS from different C. sativa L. strains, the CBDA synthase was considered as the ancestral synthase from which the THCAS evolved (Onofri et al., 2015)
en.wikipedia.org
en.wikipedia.org
en.wikipedia.org
doi: https://doi.org/10.1101/2023.08.30.555511
Posted September 01, 2023.
https://www.biorxiv.org/content/10.1101/2023.08.30.555511v1.full.pdf
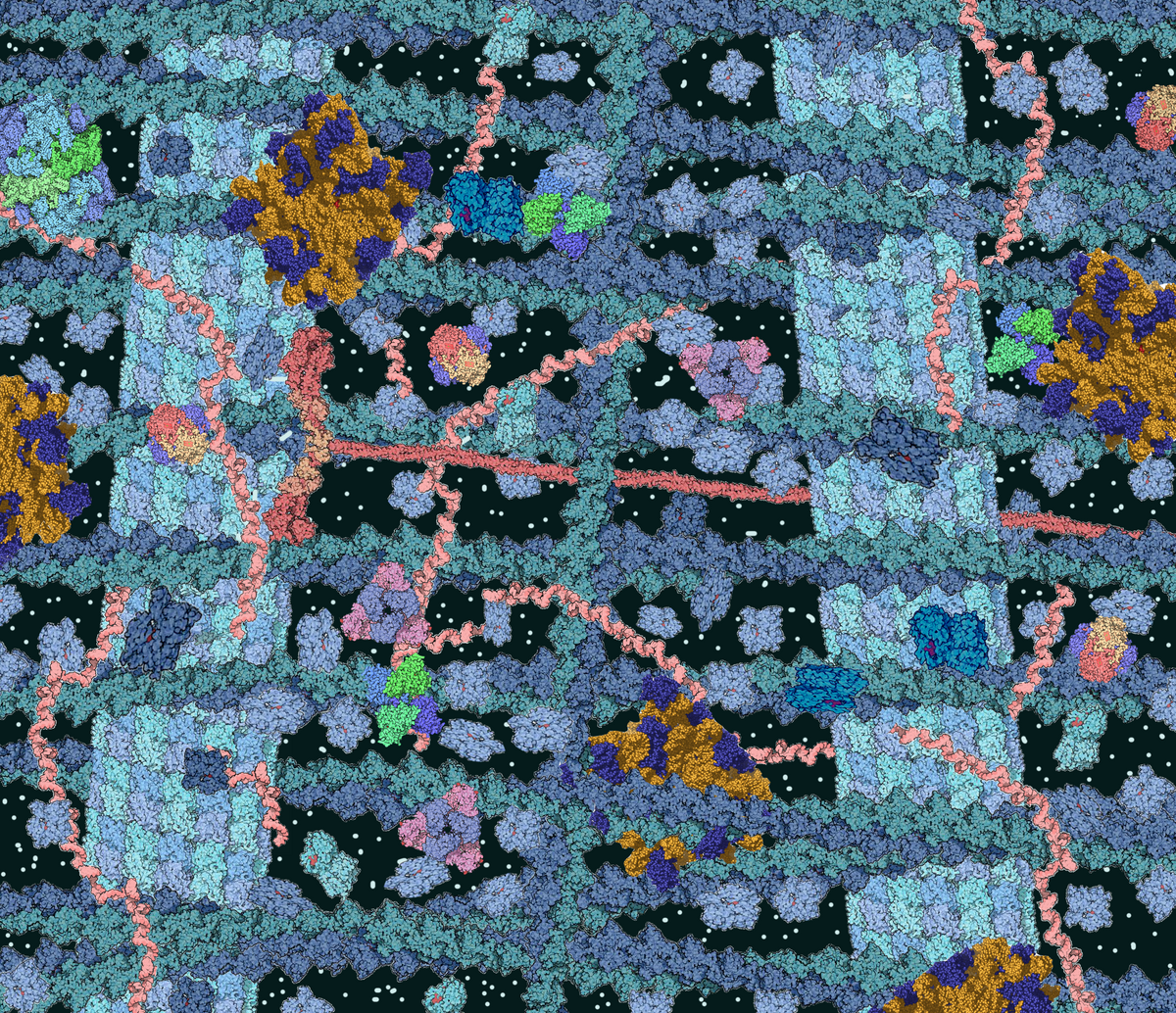
Cytosol - Wikipedia

Plastid - Wikipedia

Apoplast - Wikipedia
Natural gene variation in Cannabis sativa unveils a key region of cannabinoid synthase enzymes
View ORCID ProfileCloé Villard, Christian Bayer, Nora Pasquali Medici, Arjen C. van de Peppel, View ORCID ProfileKatarina Cankar, Francel Verstappen, View ORCID ProfileIris F. Kappers, View ORCID ProfileM. Eric Schranz, View ORCID ProfileBastian Daniel, View ORCID ProfileRobin van Velzendoi: https://doi.org/10.1101/2023.08.30.555511
Posted September 01, 2023.
https://www.biorxiv.org/content/10.1101/2023.08.30.555511v1.full.pdf
Last edited: