Im going to share my finds that I find to be so advanced they are boggling to my mind, also some insights...
I also invite you to share the same here
link your relevant threads and share knowledge together
Increased Cannabinoid Yields & Advanced Grow Teks
Everyone is most likely familiar with this, but if not heres the numbers and the science
Drought had substantial effects on cannabinoid yield, expressed as grams of cannabinoid from inflorescences per unit growing area (g·m−2). In the drought treatment, THC yield was 50% higher, THCA yield was 43% higher, CBD yield was 67% higher, and CBDA yield was 47% higher than in the control
journals.ashs.org

Limit water to maximize cannabinoid and terpene levels in cannabis production.
Dr. Deron Caplan, Ph.D. | May 2021
Figure 1. Location for leaf angle measurement to indicate degree of wilting in cannabis.
Severe drought lowers yields and kills crops, but, in moderation, drought can stimulate secondary metabolite production. Slight and infrequent drought stress combined with high solar radiation is recognized to increase essential oils in herbs and spices in places such as the Mediterranean. Fortunately, cannabis growers can also use drought to their advantage to increase the secondary metabolite content, or cannabinoids and terpenes, of their crops.
Researchers at the University of Guelph (U of G) found that a single controlled application of drought stress increased the concentration of tetrahydrocannabinol acid (THCA) and cannabidiolic acid (CBDA) by 12% and 13%, respectively, compared to a non-stressed control. Further, yield per unit growing area of THCA, CBDA, THC and CBD increased 43%, 47%, 50% and 67%, respectively.
Applying controlled drought stress may be difficult in some cultivation systems, but for those interested in experimenting (carefully), here are some tips.
Note that the wilting response to drought varies by species, and only one cultivar was used in the study. Other cultivars may wilt differently, making it important to watch your plants closely. Other drought stress indicators such as growing media moisture content may be better suited for some cultivars.
Figure 2. Visual effects of drought stress on Cannabis sativa L. ‘NC:Med (Nebula)’
Photos by Deron Caplan
Cannabinoids accumulate mostly during the flowering stage, but the timing of cannabinoid concentration varies by cultivar. It is best to collect cannabinoid content data throughout the crop to inform when to apply the stress, but this can be cost prohibitive for some growers. In most cases, a good place to start is two to three weeks before harvest and iterate from there.
In the U of G study, wilting occurred after 11 days without water. The drought stress was gradual, allowing the plants to acclimate. Keep this time frame in mind when applying drought stress in your cultivation system—you may need to adjust environmental conditions, the growing media, or the pot size to get comparable results.
Dr. Deron Caplan, PhD, is the Director of R&D at Flowr in Kelowna, B.C.
2. Caplan, D., Dixon, M. & Zheng, Y. Increasing Inflorescence Dry Weight and Cannabinoid Content in Medical Cannabis Using Controlled Drought Stress. HortScience. 54, 964–969 (2019).
3. Xu, Z., Zhou, G. & Shimizu, H. Plant responses to drought and rewatering. Plant Signal Behavior. 5, 649–54 (2010).
I also invite you to share the same here

Increased Cannabinoid Yields & Advanced Grow Teks
Everyone is most likely familiar with this, but if not heres the numbers and the science
Drought had substantial effects on cannabinoid yield, expressed as grams of cannabinoid from inflorescences per unit growing area (g·m−2). In the drought treatment, THC yield was 50% higher, THCA yield was 43% higher, CBD yield was 67% higher, and CBDA yield was 47% higher than in the control

Increasing Inflorescence Dry Weight and Cannabinoid Content in Medical Cannabis Using Controlled Drought Stress
Controlled application of drought can increase secondary metabolite concentrations in some essential oil-producing crops. To evaluate the effects of drought on cannabis (Cannabis sativa L.) inflorescence dry weight and cannabinoid content, drought stress was applied to container-grown cannabis...

3 Tips for Controlled Drought Stress
Limit water to maximize cannabinoid and terpene levels in cannabis production.
Dr. Deron Caplan, Ph.D. | May 2021

Severe drought lowers yields and kills crops, but, in moderation, drought can stimulate secondary metabolite production. Slight and infrequent drought stress combined with high solar radiation is recognized to increase essential oils in herbs and spices in places such as the Mediterranean. Fortunately, cannabis growers can also use drought to their advantage to increase the secondary metabolite content, or cannabinoids and terpenes, of their crops.
Researchers at the University of Guelph (U of G) found that a single controlled application of drought stress increased the concentration of tetrahydrocannabinol acid (THCA) and cannabidiolic acid (CBDA) by 12% and 13%, respectively, compared to a non-stressed control. Further, yield per unit growing area of THCA, CBDA, THC and CBD increased 43%, 47%, 50% and 67%, respectively.
Applying controlled drought stress may be difficult in some cultivation systems, but for those interested in experimenting (carefully), here are some tips.
Tip 1: Go slow and watch for wilting.
In the U of G study, drought stress was applied by allowing the container-grown cannabis plants to dry slowly under standard conditions. As water became limiting (around the 11-day mark), the fan leaves began to wilt and the plants were rewatered. Wilting was defined as a 50% increase in leaf angle from their normal state and measured using a handheld protractor (Fig 1).Note that the wilting response to drought varies by species, and only one cultivar was used in the study. Other cultivars may wilt differently, making it important to watch your plants closely. Other drought stress indicators such as growing media moisture content may be better suited for some cultivars.
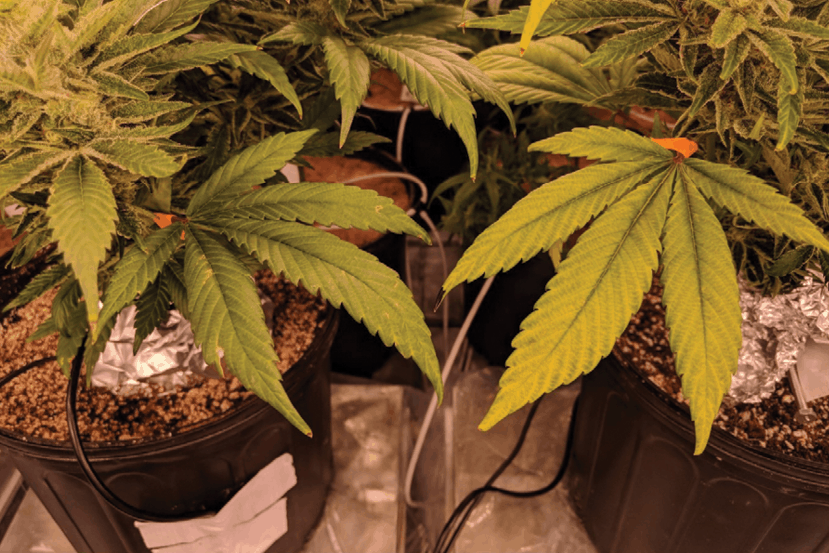
Photos by Deron Caplan
Tip 2: Apply drought stress during mid-to-late flowering.
Drought stress timing is essential to minimize yield losses and maximize the concentration of secondary metabolites. If drought is applied while the plants are growing vegetatively, you can expect a drop in yield. In the U of G study, drought was applied at week 7 in flower when vegetative growth was mostly complete.Cannabinoids accumulate mostly during the flowering stage, but the timing of cannabinoid concentration varies by cultivar. It is best to collect cannabinoid content data throughout the crop to inform when to apply the stress, but this can be cost prohibitive for some growers. In most cases, a good place to start is two to three weeks before harvest and iterate from there.
Tip 3: Start slow, and don’t overdo it.
A host of factors can influence water use in plants. For example, high heat, low humidity and high airflow can increase water usage. Additionally, a low growing media volume can decrease water availability. Both increased water usage and reduced availability can expedite drought stress symptoms.In the U of G study, wilting occurred after 11 days without water. The drought stress was gradual, allowing the plants to acclimate. Keep this time frame in mind when applying drought stress in your cultivation system—you may need to adjust environmental conditions, the growing media, or the pot size to get comparable results.
Dr. Deron Caplan, PhD, is the Director of R&D at Flowr in Kelowna, B.C.
References
1. Kleinwächter, M. & Selmar, D. New insights explain that drought stress enhances the quality of spice and medicinal plants: Potential applications. Agronomy for Sustainable Development. 35, 121–131 (2015).2. Caplan, D., Dixon, M. & Zheng, Y. Increasing Inflorescence Dry Weight and Cannabinoid Content in Medical Cannabis Using Controlled Drought Stress. HortScience. 54, 964–969 (2019).
3. Xu, Z., Zhou, G. & Shimizu, H. Plant responses to drought and rewatering. Plant Signal Behavior. 5, 649–54 (2010).
Last edited: