G
Guest
I have achieved my goal of near perfect root zones in my TAG systems so I'm on the pursuit for the ultimate TAGing Nutrient Profile.
That is the nutrient dosing regime for TAG specific growing as the accelerated growth and increased nutrient uptake demand more precisely measured nutrients IMHO.
This is my goal...If you need more clarification on my systems, their design or what the hell TAGing is..just checkout any of my signature threads as all the support information for what I'm about to vomit here is located.
I'll be basing the design of this profile on the latest and most scientific research I can find and anyone interested in lending any professional experience here ...please by all means feel free.
This is an open chalkboard of my thoughts; if you'd like to sit in and help figure this one out with me I'm sure I'd enjoy the company.
I simply request that there be respectful exchange and courtesy between contributors and no shit talking...got it? Good.
Let's begin...
I will be posting the eventual Nutrient Profile here in the first post as I learn and discover more. Currently this is to be seen as a chalkboard of ideas and theories as I'm sure I will not get it correct in the first try—this is an on going experiment...
Phase One: Inoculation of the cutting...
<http://www.growthtechnology.com/prop-rootriot.asp>
Using simiple starting medium for AM&NB innoculation this sounds perfect.
Root Riot
Made from composted organic materials, these new cubes have a great spongy texture which retains the perfect air/water ratio for healthy, rapid root growth.
Suitable for both cuttings and seeds, Root Riot consistently outperforms alternative media.
The cubes are specially inoculated with micro nutrients to nourish the young plants, and also beneficial rooting fungi to aid root development.
My Favorite:
RAPID ROOTER®
Rooting Plug
Rapid Rooter’s breakthrough technology produces a unique matrix of composted organic materials bonded together with plant-derived polymers. Rapid Rooter plugs are manufactured using a scientifically controlled process that yields large populations of beneficial microbes in the media. These naturally-occurring microbes colonize young roots, helping plants resist disease while maximizing nutrient uptake. Rapid Rooter plugs are fortified with General Hydroponic micronutrients for abundant root growth. The optimal air-to-water ratio within the plug matrix results in explosive early root growth. Use Rapid Rooter for robust early rooting that supports explosive plant growth.
Another of interest but not used:
Mighty Myco
Soluble
Premium
Mycorrhizal Inoculant
Mighty Myco Root Dip Gel Premium Mycorrhizal Inoculant is a root biostimulant that increases fruit, vegetable and flower yields, reduces transplant shock and promotes fast growth.
Contains a root dip mix of 13 carefully selected species of beneficial Endomycorrhizae and Ectomycorrhizae fungus. Mighty Myco works best when in direct contact with a plants roots. Mix 4 ounces of Mighty Myco Root Dip Gel per gallon of water. Let stand for 5 to 10 minutes, stirring occasionally. A thin slurry will form that will stick to the roots when dipped. Dip plant roots in slurry for about 5 seconds and plant in soil or medium. 4 ounces will treat between 10 and 250 plants, depending on the size of the root mass.
Active ingredients include Rhizopogon amylopogon 440,528 propagules per gram; Rhizopogon fulvigleba 440,528 propagules per gram; Rhizopogon luteolus 440,528 propagules per gram; Rhizopogon villosullus 440,528 propagules per gram; Pisolithus tinctorius 440,528 propagules per gram; Glomus aggregatum 8 propagules per gram; Glomus brazillanum 8 propagules per gram; Globus clarum 8 propagules per gram; Glomus deserticola 8 propagules per gram; Glomus intraradices 8 propagules per gram; Globus monosporum 8 propagules per gram; Glomus mosseae 8 propagules per gram; Gigaspora margarita 8 propagules per gram. Inert ingredients include 25% Polyacrylamide; 10% soluble kelp; 10% ascorbic acid; 10% humic acid; 3% Vitamin B1; 3% Glycene.
AND THE BEST LINK FOR LEARNING EVERYTHING YOU COULD EVER WANT TO KNOW ABOUT MYCOHZ
http://www.horticulturalalliance.com/Mycorrhiza_Research.asp
Phase Two: Understanding Gas Exchange
Index to this post:
Leaves
Opening stomata
Closing stomata
Density of stomata
Stomata and carbon dioxide levels
Roots and Stems
Gas Exchange in Plants
In order to carry on photosynthesis, green plants need a supply of carbon dioxide and a means of disposing of oxygen. In order to carry on cellular respiration, plant cells need oxygen and a means of disposing of carbon dioxide (just as animal cells do).
Unlike animals, plants have no specialized organs for gas exchange (with the few inevitable exceptions!). The are several reasons they can get along without them:
Each part of the plant takes care of its own gas exchange needs. Although plants have an elaborate liquid transport system, it does not participate in gas transport.
Roots, stems, and leaves respire at rates much lower than are characteristic of animals. Only during photosynthesis are large volumes of gases exchanged and each leaf is well adapted to take care of its own needs.
The distance that gases must diffuse in even a large plant is not great. Each living cell in the plant is located close to the surface. While obvious for leaves, it is also true for stems. The only living cells in the stem are organized in thin layers just beneath the bark. The cells in the interior are dead and serve only to provide mechanical support.
Most of the living cells in a plant have at least part of their surface exposed to air. The loose packing of parenchyma cells in leaves, stems, and roots provides an interconnecting system of air spaces. Gases diffuse through air several thousand times faster than through water. Once oxygen and carbon dioxide reach the network of intercellular air spaces (arrows), they diffuse rapidly through them.
Oxygen and carbon dioxide also pass through the cell wall and plasma membrane of the cell by diffusion. The diffusion of carbon dioxide may be aided by aquaporin channels inserted in the plasma membrane.
Leaves
The exchange of oxygen and carbon dioxide in the leaf (as well as the loss of water vapor in transpiration) occurs through pores called stomata (singular = stoma).
Normally stomata open when the light strikes the leaf in the morning and close during the night.
The immediate cause is a change in the turgor of the guard cells. The inner wall of each guard cell is thick and elastic. When turgor develops within the two guard cells flanking each stoma, the thin outer walls bulge out and force the inner walls into a crescent shape. This opens the stoma. When the guard cells lose turgor, the elastic inner walls regain their original shape and the stoma closes.
Time Osmotic Pressure, lb/in2
7 A.M. 212
11 A.M. 456
5 P.M. 272
12 midnight 191
The table shows the osmotic pressure measured at different times of day in typical guard cells. The osmotic pressure within the other cells of the lower epidermis remained constant at 150 lb/in2. When the osmotic pressure of the guard cells became greater than that of the surrounding cells, the stomata opened. In the evening, when the osmotic pressure of the guard cells dropped to nearly that of the surrounding cells, the stomata closed.
Opening stomata
The increase in osmotic pressure in the guard cells is caused by an uptake of potassium ions (K+). The concentration of K+ in open guard cells far exceeds that in the surrounding cells. This is how it accumulates:
Blue light is absorbed by phototropin which activates
a proton pump (an H+-ATPase) in the plasma membrane of the guard cell.
ATP, generated by the light reactions of photosynthesis, drives the pump.
As protons (H+) are pumped out of the cell, its interior becomes increasingly negative.
This attracts additional potassium ions into the cell, raising its osmotic pressure.
Closing stomata
Although open stomata are essential for photosynthesis, they also expose the plant to the risk of losing water through transpiration. Some 90% of the water taken up by a plant is lost in transpiration.
Abscisic acid (ABA) is the hormone that triggers closing of the stomata when soil water is insufficient to keep up with transpiration (which often occurs around mid-day).
The mechanism:
ABA binds to receptors at the surface of the plasma membrane of the guard cells.
The receptors activate several interconecting pathways which converge to produce a rise in pH in the cytosol transfer of Ca2+ from the vacuole to the cytosol. The increased Ca2+ in the cytosol blocks the uptake of K+ into the guard cell while the increased pH stimulates the loss of Cl- and organic ions (e.g., malate2-) from the cell.
The loss of these solutes in the cytosol reduces the osmotic pressure of the cell and thus turgor. The stomata close.
Density of stomata
The density of stomata on a leaf varies with such factors as:
the temperature, humidity, and light intensity around the plant;
and also, as it turns out, the concentration of carbon dioxide in the air around the leaves. The relationship is inverse; that is, as CO2 goes up, the number of stomata goes down, and vice versa. Some evidence:
Plants grown in an artificial atmosphere with a high level of CO2 have fewer stomata than normal.
Herbarium specimens reveal that the number of stomata in a given species has been declining over the last 200 years — the time of the industrial revolution and rising levels of CO2 in the atmosphere [View].
These data can be quantified by determining the stomatal index: the ratio of the number of stomata in a given area divided by the total number of stomata and other epidermal cells in that same area.
How does the plant determine how many stomata to produce?
It turns out that the mature leaves on the plant detect the conditions around them and send a signal (its nature still unknown) that adjusts the number of stomata that will form on the developing leaves.
Two experiments (reported by Lake et al., in Nature, 411:154, 10 May 2001):
When the mature leaves of the plant (Arabidopsis) are encased in glass tubes filled with high levels (720 ppm) of CO2, the developing leaves have fewer stomata than normal even though they are growing in normal air (360 ppm).
Conversely, when the mature leaves are given normal air (360 ppm CO2) while the shoot is exposed to high CO2 (720 ppm), the new leaves develop with the normal stomatal index.
Roots and Stems
Woody stems and mature roots are sheathed in layers of dead cork cells impregnated with suberin — a waxy, waterproof (and airproof) substance. So cork is as impervious to oxygen and carbon dioxide as it is to water.
However, the cork of both mature roots and woody stems is perforated by nonsuberized pores called lenticels. These enable oxygen to reach the intercellular spaces of the interior tissues and carbon dioxide to be released to the atmosphere.
In many annual plants, the stems are green and almost as important for photosynthesis as the leaves. These stems use stomata rather than lenticels for gas exchange.
Helpful charts:
That is the nutrient dosing regime for TAG specific growing as the accelerated growth and increased nutrient uptake demand more precisely measured nutrients IMHO.
This is my goal...If you need more clarification on my systems, their design or what the hell TAGing is..just checkout any of my signature threads as all the support information for what I'm about to vomit here is located.
I'll be basing the design of this profile on the latest and most scientific research I can find and anyone interested in lending any professional experience here ...please by all means feel free.
This is an open chalkboard of my thoughts; if you'd like to sit in and help figure this one out with me I'm sure I'd enjoy the company.
I simply request that there be respectful exchange and courtesy between contributors and no shit talking...got it? Good.
Let's begin...
I will be posting the eventual Nutrient Profile here in the first post as I learn and discover more. Currently this is to be seen as a chalkboard of ideas and theories as I'm sure I will not get it correct in the first try—this is an on going experiment...
Phase One: Inoculation of the cutting...
<http://www.growthtechnology.com/prop-rootriot.asp>
Using simiple starting medium for AM&NB innoculation this sounds perfect.
Root Riot
Made from composted organic materials, these new cubes have a great spongy texture which retains the perfect air/water ratio for healthy, rapid root growth.
Suitable for both cuttings and seeds, Root Riot consistently outperforms alternative media.
The cubes are specially inoculated with micro nutrients to nourish the young plants, and also beneficial rooting fungi to aid root development.
My Favorite:
RAPID ROOTER®
Rooting Plug
Rapid Rooter’s breakthrough technology produces a unique matrix of composted organic materials bonded together with plant-derived polymers. Rapid Rooter plugs are manufactured using a scientifically controlled process that yields large populations of beneficial microbes in the media. These naturally-occurring microbes colonize young roots, helping plants resist disease while maximizing nutrient uptake. Rapid Rooter plugs are fortified with General Hydroponic micronutrients for abundant root growth. The optimal air-to-water ratio within the plug matrix results in explosive early root growth. Use Rapid Rooter for robust early rooting that supports explosive plant growth.
Another of interest but not used:
Mighty Myco
Soluble
Premium
Mycorrhizal Inoculant
Mighty Myco Root Dip Gel Premium Mycorrhizal Inoculant is a root biostimulant that increases fruit, vegetable and flower yields, reduces transplant shock and promotes fast growth.
Contains a root dip mix of 13 carefully selected species of beneficial Endomycorrhizae and Ectomycorrhizae fungus. Mighty Myco works best when in direct contact with a plants roots. Mix 4 ounces of Mighty Myco Root Dip Gel per gallon of water. Let stand for 5 to 10 minutes, stirring occasionally. A thin slurry will form that will stick to the roots when dipped. Dip plant roots in slurry for about 5 seconds and plant in soil or medium. 4 ounces will treat between 10 and 250 plants, depending on the size of the root mass.
Active ingredients include Rhizopogon amylopogon 440,528 propagules per gram; Rhizopogon fulvigleba 440,528 propagules per gram; Rhizopogon luteolus 440,528 propagules per gram; Rhizopogon villosullus 440,528 propagules per gram; Pisolithus tinctorius 440,528 propagules per gram; Glomus aggregatum 8 propagules per gram; Glomus brazillanum 8 propagules per gram; Globus clarum 8 propagules per gram; Glomus deserticola 8 propagules per gram; Glomus intraradices 8 propagules per gram; Globus monosporum 8 propagules per gram; Glomus mosseae 8 propagules per gram; Gigaspora margarita 8 propagules per gram. Inert ingredients include 25% Polyacrylamide; 10% soluble kelp; 10% ascorbic acid; 10% humic acid; 3% Vitamin B1; 3% Glycene.
AND THE BEST LINK FOR LEARNING EVERYTHING YOU COULD EVER WANT TO KNOW ABOUT MYCOHZ
http://www.horticulturalalliance.com/Mycorrhiza_Research.asp
Phase Two: Understanding Gas Exchange
Index to this post:
Leaves
Opening stomata
Closing stomata
Density of stomata
Stomata and carbon dioxide levels
Roots and Stems
Gas Exchange in Plants
In order to carry on photosynthesis, green plants need a supply of carbon dioxide and a means of disposing of oxygen. In order to carry on cellular respiration, plant cells need oxygen and a means of disposing of carbon dioxide (just as animal cells do).
Unlike animals, plants have no specialized organs for gas exchange (with the few inevitable exceptions!). The are several reasons they can get along without them:
Each part of the plant takes care of its own gas exchange needs. Although plants have an elaborate liquid transport system, it does not participate in gas transport.
Roots, stems, and leaves respire at rates much lower than are characteristic of animals. Only during photosynthesis are large volumes of gases exchanged and each leaf is well adapted to take care of its own needs.
The distance that gases must diffuse in even a large plant is not great. Each living cell in the plant is located close to the surface. While obvious for leaves, it is also true for stems. The only living cells in the stem are organized in thin layers just beneath the bark. The cells in the interior are dead and serve only to provide mechanical support.
Most of the living cells in a plant have at least part of their surface exposed to air. The loose packing of parenchyma cells in leaves, stems, and roots provides an interconnecting system of air spaces. Gases diffuse through air several thousand times faster than through water. Once oxygen and carbon dioxide reach the network of intercellular air spaces (arrows), they diffuse rapidly through them.
Oxygen and carbon dioxide also pass through the cell wall and plasma membrane of the cell by diffusion. The diffusion of carbon dioxide may be aided by aquaporin channels inserted in the plasma membrane.
Leaves
The exchange of oxygen and carbon dioxide in the leaf (as well as the loss of water vapor in transpiration) occurs through pores called stomata (singular = stoma).
Normally stomata open when the light strikes the leaf in the morning and close during the night.
The immediate cause is a change in the turgor of the guard cells. The inner wall of each guard cell is thick and elastic. When turgor develops within the two guard cells flanking each stoma, the thin outer walls bulge out and force the inner walls into a crescent shape. This opens the stoma. When the guard cells lose turgor, the elastic inner walls regain their original shape and the stoma closes.
Time Osmotic Pressure, lb/in2
7 A.M. 212
11 A.M. 456
5 P.M. 272
12 midnight 191
The table shows the osmotic pressure measured at different times of day in typical guard cells. The osmotic pressure within the other cells of the lower epidermis remained constant at 150 lb/in2. When the osmotic pressure of the guard cells became greater than that of the surrounding cells, the stomata opened. In the evening, when the osmotic pressure of the guard cells dropped to nearly that of the surrounding cells, the stomata closed.
Opening stomata
The increase in osmotic pressure in the guard cells is caused by an uptake of potassium ions (K+). The concentration of K+ in open guard cells far exceeds that in the surrounding cells. This is how it accumulates:
Blue light is absorbed by phototropin which activates
a proton pump (an H+-ATPase) in the plasma membrane of the guard cell.
ATP, generated by the light reactions of photosynthesis, drives the pump.
As protons (H+) are pumped out of the cell, its interior becomes increasingly negative.
This attracts additional potassium ions into the cell, raising its osmotic pressure.
Closing stomata
Although open stomata are essential for photosynthesis, they also expose the plant to the risk of losing water through transpiration. Some 90% of the water taken up by a plant is lost in transpiration.
Abscisic acid (ABA) is the hormone that triggers closing of the stomata when soil water is insufficient to keep up with transpiration (which often occurs around mid-day).
The mechanism:
ABA binds to receptors at the surface of the plasma membrane of the guard cells.
The receptors activate several interconecting pathways which converge to produce a rise in pH in the cytosol transfer of Ca2+ from the vacuole to the cytosol. The increased Ca2+ in the cytosol blocks the uptake of K+ into the guard cell while the increased pH stimulates the loss of Cl- and organic ions (e.g., malate2-) from the cell.
The loss of these solutes in the cytosol reduces the osmotic pressure of the cell and thus turgor. The stomata close.
Density of stomata
The density of stomata on a leaf varies with such factors as:
the temperature, humidity, and light intensity around the plant;
and also, as it turns out, the concentration of carbon dioxide in the air around the leaves. The relationship is inverse; that is, as CO2 goes up, the number of stomata goes down, and vice versa. Some evidence:
Plants grown in an artificial atmosphere with a high level of CO2 have fewer stomata than normal.
Herbarium specimens reveal that the number of stomata in a given species has been declining over the last 200 years — the time of the industrial revolution and rising levels of CO2 in the atmosphere [View].
These data can be quantified by determining the stomatal index: the ratio of the number of stomata in a given area divided by the total number of stomata and other epidermal cells in that same area.
How does the plant determine how many stomata to produce?
It turns out that the mature leaves on the plant detect the conditions around them and send a signal (its nature still unknown) that adjusts the number of stomata that will form on the developing leaves.
Two experiments (reported by Lake et al., in Nature, 411:154, 10 May 2001):
When the mature leaves of the plant (Arabidopsis) are encased in glass tubes filled with high levels (720 ppm) of CO2, the developing leaves have fewer stomata than normal even though they are growing in normal air (360 ppm).
Conversely, when the mature leaves are given normal air (360 ppm CO2) while the shoot is exposed to high CO2 (720 ppm), the new leaves develop with the normal stomatal index.
Roots and Stems
Woody stems and mature roots are sheathed in layers of dead cork cells impregnated with suberin — a waxy, waterproof (and airproof) substance. So cork is as impervious to oxygen and carbon dioxide as it is to water.
However, the cork of both mature roots and woody stems is perforated by nonsuberized pores called lenticels. These enable oxygen to reach the intercellular spaces of the interior tissues and carbon dioxide to be released to the atmosphere.
In many annual plants, the stems are green and almost as important for photosynthesis as the leaves. These stems use stomata rather than lenticels for gas exchange.
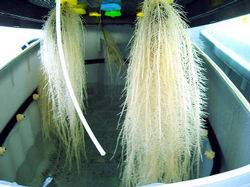

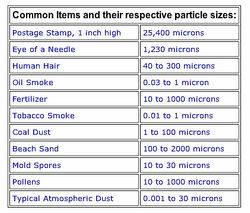
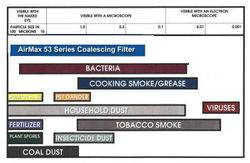
Helpful charts:
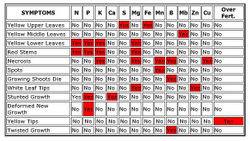
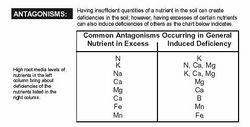

Last edited: